The Future Of Energy Storage On Both Sides Of The Meter
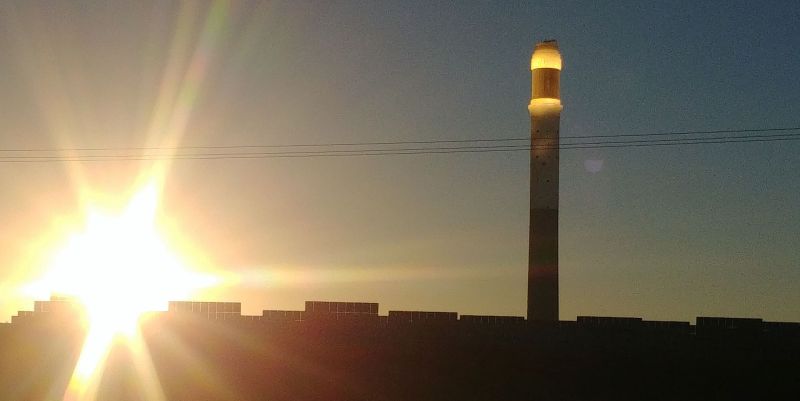
That energy storage is a hot topic is hardly a surprise to anyone these days. Even so, energy storage can take a lot of different forms, some of which are more relevant to the utility provider (like grid-level storage), while others are relevant to business and home owners (e.g. whole-house storage), and yet other technologies live in this tense zone between utility and personal interest, such as (electric) vehicle-to-grid.
For utilities a lot of noise is being made about shiny new technologies, such as hydrogen-based storage, while home- and business owners are pondering on the benefits of relying solely on the utility’s generosity with feed-in tariffs, versus charging a big battery from the solar panels on the roof and using the produced power themselves. Ultimately the questions here are which technologies will indeed live up to their promises, and which a home owner may want to invest in.
Dealing With A Changing Grid
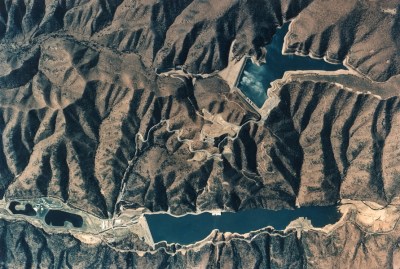
For many decades the electrical grid has been a relatively straightforward system with dispatchable generators and consumers, with the former producing when required and the latter consuming and a bit of on-grid storage to smooth over the bumps and valleys. The biggest change that has been occurring over the past decades has been the introduction of electrical generators that only produce electricity when weather and other conditions are just right.
The result of this has been that the peaks got a lot taller and the valleys a lot deeper. While curtailment of unnecessary (excess) power would be a potential solution, a popular idea is to use as much of this excess power as possible at a later point by storing it somehow. At this point in time, most grid-level energy storage is provided by compressed air energy storage (CAES) and pumped hydro storage (PHS). What these two technologies have in common is that they both have a relatively low energy density by volume, but make up by that through having a lot of volume.
In the case of the second-largest PHS system, the Bath County Pumped Storage Station in Virginia, its upper reservoir has a capacity of 43,000,000 m3 (35,599 acre-ft), which provides it with enough gravity potential to provide power for 11 hours at around 3 GW, for a total of 24 GWh. With a round-trip efficiency of 79%, it provides important storage for PJM Interconnection, to buffer energy and take the stress off transmission interconnects between parts of the grid.
For CAES there are not nearly as many installations as there are of PHS installations, mostly due to the complexity of finding subterranean caverns with the right (airtight) properties. Because of this, there are only two CAES installations in operation in the world today: the McIntosh, Alabama (USA) and Huntorf, Elsfleth (Germany) CAES plants. The former has a capacity of 2,860 MWh, the latter 870 MWh.
As a result of the diabatic process used with these CAES plants, the compression process creates waste heat, and subsequent expansion requires input of heat. In the case of these existing plants, natural gas plants are used for this, which in the case of the McIntosh CAES plant results in an overall system efficiency of 27%, up to over 40% when combined with energy recovery mechanisms.
For PHS the number of potential new sites where such storage sites could be economically constructed aren’t too many, which has led to the focus on new technologies that could provide PHS-like storage, without the logistical and situational complications.
Hydrogen Storage
The so-called ‘hydrogen economy‘ has its roots in the 1970s, when the term was coined by John Bockris during a talk at General Motors. The main driving idea behind it is that hydrogen can be used to decarbonize many aspects of industrial processes, as well as to create ammonia (NH3), which forms an essential element of fertilizer. It’s also been suggested to use ammonia as an intermediate form before converting back to hydrogen.
At this point in time natural gas (NG, mostly fossil methane) provides most of the hydrogen and ammonia used. As a result, the use of excess electricity to create hydrogen via electrolysis has been suggested as a source instead. None of this has been developed into large-scale projects yet, however. This comes alongside trials to mix hydrogen into NG, up to a certain percentage. Large amounts of hydrogen in NG infrastructure can lead to issues such as metal embrittlement, due to the hydrogen diffusing through pipe walls.
To store hydrogen, for later use (e.g. time-shifting large amounts of energy), currently mature technologies are compression and liquefying. Complications here are the immediate energy loss from electrolysis (~20-30%), the losses of compression or cooling down the hydrogen gas, the leakage from storage containers, and if conversion back to electricity is desired, the 40-60% efficiency of a hydrogen fuel cell (HFC).
Everything taken together, the round-trip efficiency of a hydrogen-based storage system for the grid would be between 18 – 46%, according to studies. This would be a major drop in efficiency compared to PHS and CAES systems, while adding more complexity and the potential hazards of handling cryogenic liquids and highly inflammable gases. This makes such a system a very hard sell. When the hydrogen is to be used immediately, the question is also whether it cannot be produced more efficiently through e.g. radiolysis.
Thermal Energy Storage
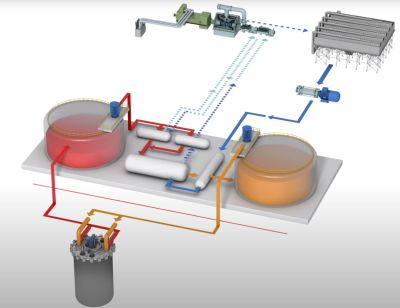
Comparatively, thermal energy storage (TES) is significantly more efficient and straightforward. It’s seeing significant use in the form of geothermal heat pumps which can cool and heat buildings rather efficiently. In an industrial setting, molten salt storage is a common type of sensible heat storage, the concept of which is behind storage heaters, which many private homes use.
With concentrated solar power (CSP) plants, molten salt is often used to store the heat from the solar rays, after which the heated salt can be used to generate steam for use with a conventional steam turbine generator. This is also the operating principle behind the TerraPower Natrium Generation IV reactor, which uses the heat from nuclear fission to heat the salt. Because of the slow heat loss, and high efficiency of such a heat transfer system, it can be used to generate electricity or heat, even after storing the molten salt and not using its thermal energy for a week.
A 400 MWh capacity system would need a tank of about 9 m tall and 24 m in diameter, using nothing but conventional materials that would be safe to handle (when not hot). Compared to the complexities of PHS, CAES and especially hydrogen-based systems, TES could end up playing a big role for energy storage. Perhaps unsurprisingly, the Ouarzazate CSP plant in Morocco is the largest energy storage plant on account of its molten salt storage at 3,005 MWh.
Keeping Things Simple
Even if PHS and CAES capacity is unlikely to be expanded significantly any more, there would seem to be still a number of technologies that utility providers can look at to expand storage capacity, even without resorting to electrochemical batteries. This also gives some hints as to what might make sense for private home and business owners.
Since on-grid storage is unlikely to see massive expansion, there is little incentive for grid operators to motivate the feed-in of intermittent power from solar and wind power. When there is the option of using locally installed PV solar panels as well solar water heating systems (sometimes combined in the same panel) to charge up a battery installed inside the same building and heat up water, the costs of this system are highly predictable, and compensated by the electricity (and natural gas) not used from the utility provider.
With the many uncertainties in the energy market and the current world economy it’s hard to say what the coming years will bring, but sticking with proven systems, and aiming for local consumption for small producers might be just the ticket.
[Heading image: Noor III Solar Tower of the Ouarzazate Power Station, at dusk. (Credit: Marc Lacoste) ]
Post a Comment