Fueling Up For Fusion: MAST’s Super-X, JET’s Deuterium-Tritium Experiments For ITER, And More
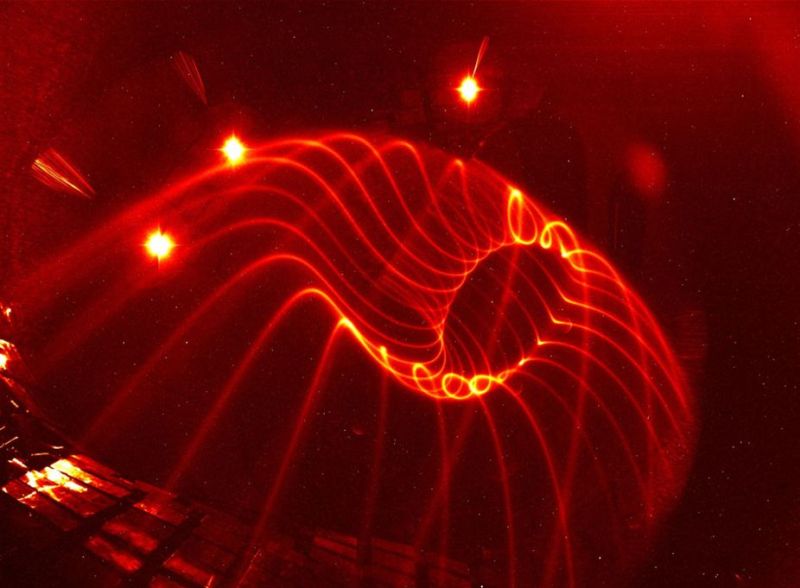
We’ve had nuclear fission reactors in operation all over the world for ages, but nuclear fusion always seems to be a decade or two away. While one cannot predict when we’ll reach the goal of sustained nuclear fusion, the cutting edge in test hardware is advancing at a rapid pace that makes us optimistic. Beginning as soon as this month and extending over a few years, we’re living through a very exciting time for nuclear fusion and plasma physics.
The Mega Ampere Spherical Tokamak (MAST) got a big upgrade to test a new cooled divertor design. JET (Joint European Torus) will be testing the deuterium-tritium fuel mixture that will be powering the ITER (the research project whose name began as an acronym for International Thermonuclear Experimental Reactor but has since been changed to just ITER). And the Wendelstein 7-X stellarator is coming back online with upgraded cooled divertors by next year.
Here the MAST Upgrade’s Super-X divertors have so far shown a ten-fold decrease in the temperature which the divertor is exposed to while carrying thermal energy out of the tokamak reactor. This means a divertor design and ultimately a fusion reactor that will last longer between maintenance sessions. On the stellarator side of things, Wendelstein 7-X’s new divertors may allow it to demonstrate the first continuous operation of a stellarator fusion reactor. Meanwhile, JET’s fuel experiments should allow us to test the deuterium-tritium fuel while ITER is working towards first plasma by 2025.
Nuclear Physics on Hard Mode
The reason why we figured out how to use nuclear fission in a commercial setting so quickly can be essentially summarized as it being a process that easily happens by itself. The difficulty with nuclear fission is more with controlling the nuclear chain reaction, requiring a balance between neutron moderation and absorption in the case of thermal neutrons and the common light water reactors (LWRs).
On the other hand, nuclear fusion is a completely different game. Instead of using some of the heaviest isotopes around and lobbing some neutrons at them to convert a part of them to energy, nuclear fusion uses the lightest isotopes (hydrogen isotopes) possible and attempts to fuse them. This is obviously not a process that easily happens by itself, unless you happen to take a very large amount of hydrogen and allow immense gravitational pressure to kickstart the fusion process, as is the case in stars.
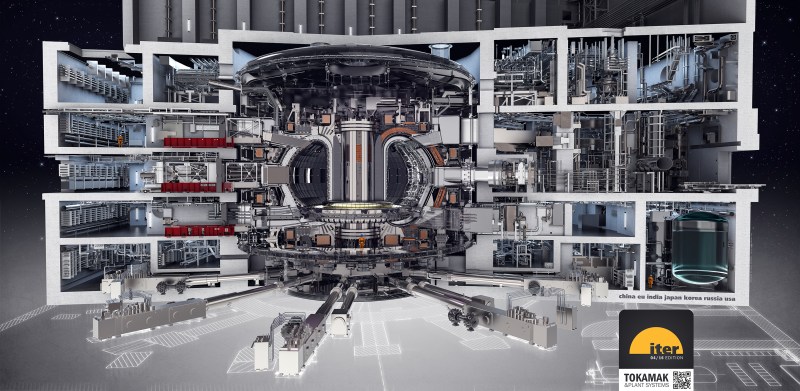
While on Earth we can find the remnants of natural fission reactors today from a time when there was more natural uranium-235 around, fusion has obviously remained limited to stars, including our Sun. As a result, the only way that we are going to accomplish nuclear fusion in a sustained reaction on Earth is by creating the right pressure, temperature, and other conditions that will allow hydrogen isotopes to conquer their fear of the Coulomb barrier and fuse so that they can release a lot of energy in the process.
This is in short the reason why it has taken over a hundred years now since the first discovery of the nuclear fusion process. A hundred years of careful experimentation and the use of new materials and analytical technologies to improve our understanding of high-temperature plasma physics and our ability to create and sustain the conditions for fusion in such a contained plasma.
Going for Break-Even
An essential metric in determining how well a nuclear fusion reactor design works is through the Q-factor. This is the ratio between input and output energy. In order for a fusion reactor to start operating, it has to literally warm up which costs energy. Sustaining these operating temperatures of 100 million degrees Celsius or more requires a certain energy input; preventing the cooling of the plasma is one area of the research.
Ideally the reaction becomes self-sustaining, in which the fusion reaction provides the energy required for sustaining the plasma temperature and fusion reaction. Yet even with some input energy required, the Q factor must be over 1 to result in any actual energy output. This basically means more energy output than what is required by the reactor’s magnetic field generator and other elements.
With next month’s JET deuterium-tritium fuel experiments the goal is to see whether the energy output can be boosted through these fuel mixtures. Currently JET has achieved a Q of 0.67 which it hopes to improve on. It’s projected that ITER’s successor (DEMO) would need to hit a Q of at least 25 to make the economic case for fusion reactors. ITER is slated to hit a Q of 10.
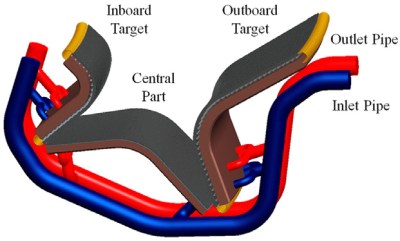
The Super-X divertors that are being tested by MAST Upgrade could be helpful here with improving efficiency of the fusion reaction. One of the purposes of these divertors is to purify the plasma by removing unwanted elements, such as the helium atoms that are produced as a result of the fusion process. Keeping the plasma stable and pure are two essential factors in making a fusion reactor work efficiently.
Even so, the magnetic fields in these tokamak designs that contain the plasma continue to form a source of constant headaches, as the plasma isn’t content to keep moving in simple, predictable patterns. Between kink instability and banana orbits (neoclassical transport) the plasma tends to show sudden movements away from the center of the magnetic field. Effectively dealing with this is still a major concern, also because it can result in fuel being lost as it escapes the magnetic field.
This is one area where the stellarator design may have a leg up on the tokamak design.
Cool Flow
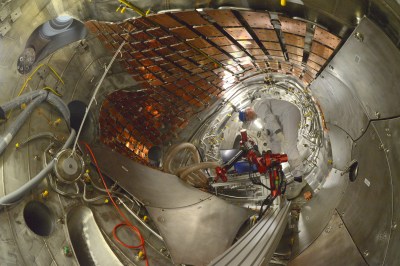
Compared to the simple, round tokamak design, stellarators seem quaint at the best of times, but this is a result of these fusion reactors following the plasma behavior instead of seeking to control it. The almost organic-looking magnetic coil configuration is the result of modeling of the plasma to find a configuration which essentially works with the super-heated plasma instead of against it.
As a modern stellarator design, the Wendelstein 7-X (W7-X) incorporates all of the know-how about these complex devices. Since first plasma in 2015, W7-X’s performance has been essentially flawless, so that in 2018 the next round of upgrades began. This involves among other changes the installation of the water-cooled divertors.
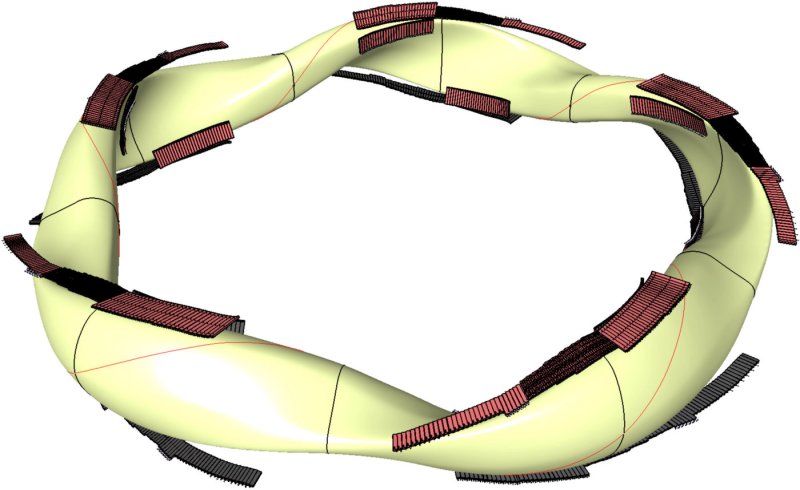
With the uncooled divertors operational time was limited, but the goal with the new and improved W7-X is to perform a continuous run for up to 30 minutes. Considering that for tokamak designs a run-time for more than a minute is considered a pretty good length, this would be a major achievement.
Although the W7-X is not intended to produce more power than is put into it, it serves the important function of demonstrating that the stellarator fusion reactor design is at least as capable as the tokamak design. This makes the next series of experiments rather exciting. Unfortunately, due to the SARS-CoV-2 pandemic that has kept the world occupied for over a year now, the W7-X upgrades have been delayed, so that the resumption of experiments likely won’t take place until next year.
A Lot to Look Forward To
Much about nuclear fusion reactors has been an intense and rather unforgiving course in plasma physics and other elementary areas of physics. When the optimistic assumption that was made in the 1950s about nuclear fusion being ‘only a few years away’ was snuffed out harshly when Z-pinch designs proved to be unworkable, the field seemed to fade away in the public’s opinion for many years.
Fast-forward countless scientific papers over many decades later and it appears that we may now cautiously consider ourselves to be on the cusp of truly workable nuclear fusion reactors. While it’s not quite a ‘within the next few years’ kind of deal, ITER and related tokamaks such as China’s CFETR give us hope for tokamak-style reactors. Meanwhile, W7-X’s progress forms an alternate approach that may work as well, worse or better than tokamaks.
With ITER’s deuterium-tritium fuel plasma still at least fourteen years away, we have a lot to look forward to with these current tokamak experiments at the JET and MAST laboratories. When we get the first experimental results from W7-X in the coming years, perhaps they will be promising enough to kickstart a stellarator version of ITER. Whatever the case may be, this decade looks to be one in which nuclear fusion is slowly shuffling back into the limelight.
Post a Comment